Although mechanical seals are used in all sorts of dirty fluids, it is well established that reliability is best when the fluid is clean. Therefore, there is a great incentive to provide a clean fluid flush for seals in dirty services. Piping Plan 31 is intended to clean up a dirty fluid to provide a clean flush to the seals. Frankly, I don’t particularly care for Plan 31 and have rarely used or recommended it.
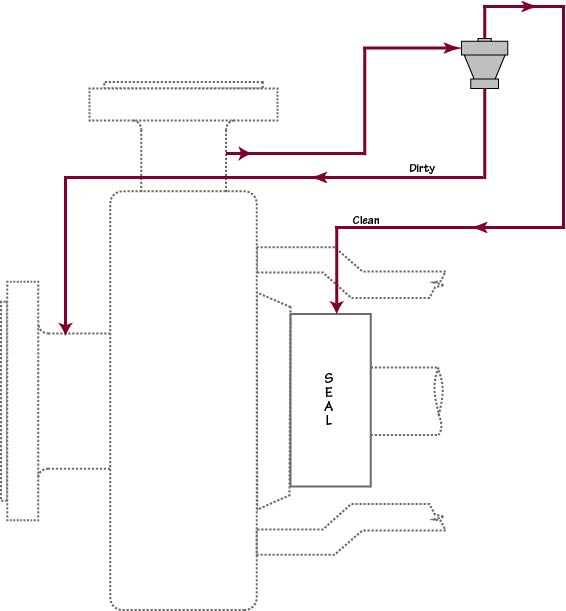
Recently I was asked a question about Plan 31 and realized that my description in SealFAQs was somewhat lacking so I’ll be revising and expanding that page.
Plan 31 relies on a “cyclone separator”, sometimes called an “abrasive separator”, or “hydrocyclone” to remove the dirt from the process fluid. I’ve always preferred the term “cyclone separator” because it gives me a mental image of how the separation works.
In Plan 31, the pumped (dirty) product flows to a cyclone separator from the discharge of the pump. The cyclone separator, uh, separates the pumpage into clean and dirty streams by using centrifugal force. The clean fluid stream is routed out the top of the separator and into the seal chamber while the now concentrated dirty fluid stream is routed back to pump suction. Roughly speaking, the clean and dirty steams are approximately equal in flow rate; that is, a 50/50 split.
The late Robert Watkins of Chevron absolutely hated Plan 31. More specifically, he hated cyclone separators. One day Robert and I were teaching a seals class when, to my astonishment, I realized that Robert was saying that a cyclone separator should be purchased for every pump! He then laughed and said “… and it should be fastened to the motor with baling wire!” His theory was that, after having bought a cyclone separator for each pump, the seal salesman would leave him alone. Plus, he could look everyone in the eye and say “Yes, we have a cyclone separator at that pump.”
I’ve removed more Plan 31 systems than I’ve added but Plan 31 can work. The only one that I’ve personally known to solve a problem was in a river water pump. The water had so much sand in it that sand was deposited in the seal chamber and could be seen when the pump was disassembled. Plan 31 was added and essentially solved the sand problem. My guideline became “If dirt is being deposited in the seal chamber then change to Plan 31.” I’ve also seen demos and lab tests showing that Plan 31 can work but the key is to know and control the differential pressures.
Most people do not realize that the cyclone separator in Plan 31 works best when the clean and dirty outlets – meaning the outlets at the separator itself — are at about the same pressure. For this reason, Plan 31 really needs pressure gauges, orifices and/or control valves. However, neither the API schematics nor my own illustrations show these gauges, orifice and valves. Here’s a new illustration that I’ll be using.
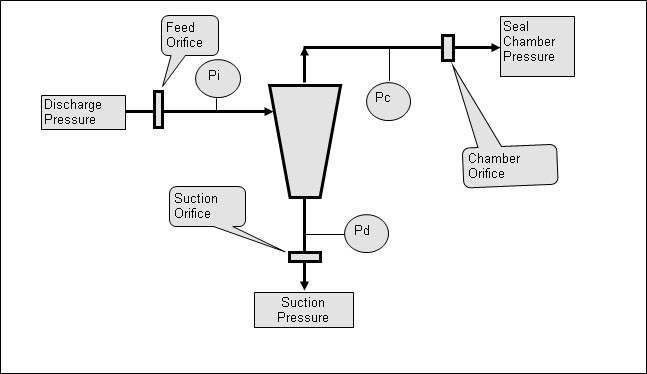
A cyclone separator needs at least a 15 psi differential to work well. However, if there is too much total differential pressure across the separator, then the centrifugal “streamlines” inside the separator become confused/turbulent and the separator does not work well. Typically, a maximum total differential pressure of around 200 psi across the separator is recommended. The orifices are used to set and control the differential pressure across the separator.
Long ago, I was called on to troubleshoot a pump/seal having a Plan 41 (Plan 31 with a heat exchanger). To my surprise, I discovered that there was no flow to the seal. Instead, flow was from the seal chamber to the cyclone and then to pump suction. (I found this by feeling around the heat exchanger for differential temperatures.)
Even when designed and installed properly, Plan 31 does not remove all the solids from the pumpage (dirty) stream. For example, the performance might be stated as something like “cyclone separator will remove 90% of all heavy solids having a diameter of 10 microns or larger.” By “heavy” is meant that the solids should have a density more than twice that of the liquid. Also, the concentration of solids should be less than 10%. In addition, the cyclone separator does not do well in viscous fluids.
So I don’t particularly like or recommend Plan 31.